Soil Contamination and Human Health
Antibiotic-resistant bacteria
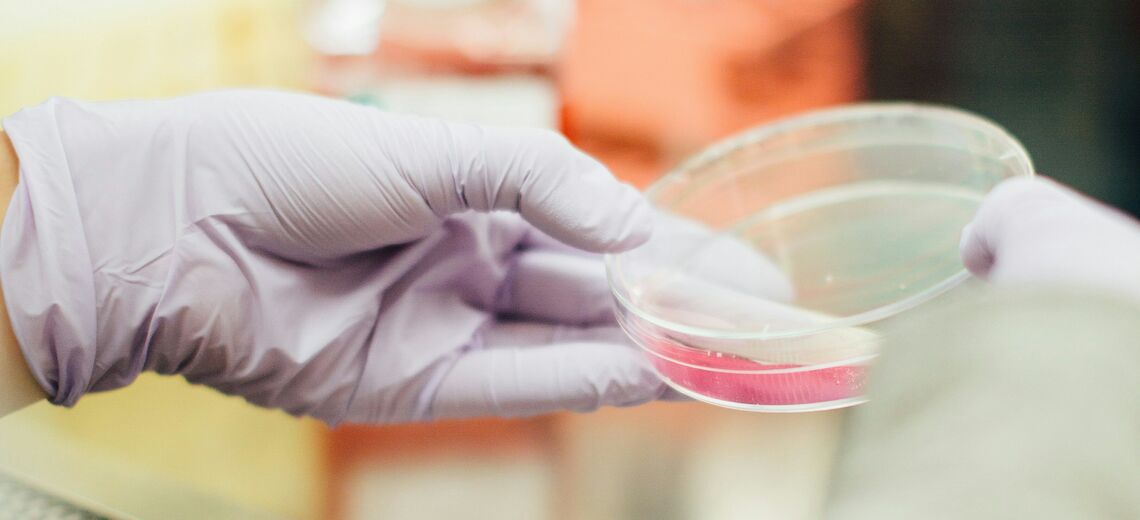
Resistance development against chemical agents is a natural phenomenon, yet the presence of antibiotic residues in agricultural soils can accelerate both the development and proliferation of resistant bacteria strains (Gudda et al., 2020).
It is well acknowledged that the expansion of resistances against antibiotics poses a growing risk to public health (He, Yan, Chen, 2020). However, the emergence and spread of antibiotic-resistant bacteria and antibiotic-resistant genes from agricultural systems and their contribution to the resistance of human pathogens are complex processes that are not yet well understood (Jechalke et al., 2014).
Studies have reported that low level concentrations of antibiotics (below minimum inhibitory concentrations), which can be found in food crops, might impact bacterial resistance selection, contributing to resistance enrichment (Gudda et al., 2020).
Agricultural soils are natural reservoirs of antibiotics and therefore also of antibiotic-resistant bacteria and genes (ARBG) (Wang et al., 2020). Elevated levels of ARBG in agricultural soils are mainly caused by the continuous application of manure from animals that were given antibiotics for therapeutic or non-therapeutic purposes, the application of contaminated sewage sludge or biosolids, or irrigation with contaminated water or reclaimed wastewater (Wang et al., 2020; Pan et al. 2017). Additionally, some antibiotics are used as pesticides in crop production (Iwu et al., 2020).
Animal manure is considered a major source of ARBG in soils (Chen et al., 2016), as more than 50% of antibiotics in Europe are administered to livestock (Münch et al., 2020). 30–90% of these antibiotics are estimated to be excreted again largely non metabolized by the animals, resulting in commonly high residual antibiotic concentrations in animal manure (Marshall et al., 2011; Wang et al., 2015; Wang et al., 2021).
The risk of soil contamination from this source is increasing, as the global amount of manure application to agricultural soils is persistently growing due to a rising livestock production (Kabelitz et al., 2020). Additionally, the utilization of manure as an organic fertilizer is promoted by the EU, both in the context of expanding organic food production and as part of a circular economy (Tadić et al., 2021). This could further exert selection pressure for antibiotic resistances (Wang et al., 2015).
Soils may represent a reservoir of both intrinsic and acquired antibiotic-resistant genes (Wang et al., 2021). When introduced into agricultural soils, antibiotics may be subjected to different processes, including transformation, sorption, plant uptake, removal with runoff, or transport into groundwater (Balseiro-Romero et al., 2018; Cycoń et al., 2019; Du & Liu, 2011). In addition, other contaminants discharged by human activities (e.g., heavy metals, persistent organic pollutants, microplastics, pesticides) may contribute to the selection and dissemination of resistances against antibiotics (Wang et al., 2020; Wang et al., 2021; Gorovtsov et al., 2018), making it very difficult to predict the evolution of antibiotic-resistant bacteria in polluted soils (Balseiro-Romero et al., 2018).
Fertilization with manure that contains antibiotic-resistant bacteria significantly contributes to airborne particular matter emergence (Kabelitz et al., 2020). Wind erosion, particularly when accelerated by agricultural operations such as tillage, further contributes to the emissions of antibiotic-resistant bacteria to the atmosphere (Münch et al., 2020; Kabelitz et al., 2020). These dynamic processes, which define the spatial–temporal distribution and thus human health risks of antibiotics, are closely interrelated (Du & Liu, 2011) and influenced by soil properties, climate factors, and physicochemical properties of antibiotics (Pan & Chu, 2017; Iwu et al., 2020; Cycoń et al., 2019; Zalewska et al., 2021).
As antibiotic-resistant bacteria and antibiotic-resistant genes (ARGB) can be absorbed by plants or can be found on their surface, the food chain has been suggested as a major pathway for human exposure (He et al., 2020; Wang et al., 2021). Ingestion of plants carrying ARBG may alter the human microbiome and promote the emergence of and selection for resistant bacteria inhabiting the human body (Marshall & Levy, 2011; Ben et al., 2018). This could reduce the efficiency of therapeutic antibiotics, increase the duration and seriousness of infections, and ultimately result in treatment failures or even mortality (Ben et al., 2018; Economou et al., 2015).
Another potential pathway for human exposure is the atmospheric dispersal of ARBG via dust or particulate matter that arise from agricultural soils treated with contaminated organic fertilizers such as manure or sewage sludge (Münch et al., 2020; Kabelitz et al., 2020). The risk of respiratory viral–bacterial coinfection should be considered in this regard, as with acquired antibiotic resistances they are expected to be more difficult to treat (Jin et al., 2021). However, understanding how transmitted antibiotic-resistant bacteria influence their counterparts in the respiratory system and exchange antibiotic resistant genes upon inhalation is insufficiently understood (Jin et al., 2021; Zhang et al., 2019).
Overall, the emergence and spread of antibiotic resistant bacteria and antibiotic resistant genes from agricultural systems and their contribution to the resistance of human pathogens are complex processes that are not yet fully understood (Jechalke et al., 2014). More research is needed to quantify the risk of human exposure to antibiotics, antibiotic-resistant bacteria, and antibiotic-resistant genes (Iwu et al., 2020), particularly the risk of long-term consumption of low doses (Pan & Chu, 2017; Tadić et al., 2021). Additionally, little knowledge exists about antibiotic transformation products, their metabolites, mixtures, and associated resistance selection (Gudda et al., 2020).
In soils contaminated with antibiotics, root uptake is considered an important route of exposure for plants. Studies indicate the accumulation and selection of antibiotic-resistant genes in different plant tissues such as leaves, stem, or fruits (Pan & Chu, 2017; Wang et al., 2021). This suggests that plants may contribute to the acquisition of antibiotics resistances in humans (Du & Liu, 2011). Moreover, residues of antibiotics may affect seed germination, photosynthesis, plant development, and productivity (Balseiro-Romero et al., 2018; Pan & Chu, 2017; Zhao et al., 2018; Matthews & Reinhold, 2013). However, depending mainly on the concentration of antibiotics, both inhibitory and stimulatory effects have been reported (Pan & Chu, 2017; Matthews & Reinhold, 2013).
Antibiotics may change the abundance of soil microorganisms, the microbial biomass, as well as overall microbial and enzyme activity (Cycoń et al., 2019), which in turn may disturb various soil processes mediated by microorganisms (Jechalke et al., 2014; Cycoń et al., 2019). They can significantly change the structure of soil microbial communities (Wang et al., 2021), particularly bacteria (Du & Liu, 2011), generating an environment for selection and outgrowth of antibiotic-resistant bacteria, which results in changes of entire microbial populations to antibiotic sensitivity (Cycoń et al., 2019). Studies suggest that low concentrations of antibiotics (below the minimum inhibitory concentration) are able to induce genetic changes in bacterial genomes, even between distantly related bacterial species (Cycoń et al., 2019).
Abdel-Rahman, G.N.-E. (2021) Heavy metals, definition, sources of food contamination, incidence, impacts and remediation: A literature review with recent updates. Egypt. J. Chem. 65, 1419–1437.
Balseiro-Romero, M.; Baveye, P.C. (2018) Book Review: Soil Pollution: A Hidden Danger Beneath our Feet. Front. Environ. Sci. 6, 130.
Ben, Y.; Fu, C.; Hu, M.; Liu, L.; Wong, M.H.; Zheng, C. (2018) Human health risk assessment of antibiotic resistance associated with antibiotic residues in the environment: A review. Environ. Res. 169, 483–493.
Chen, Q.; An, X.; Li, H.; Su, J.; Ma, Y.; Zhu, Y.-G. (2016) Long-term field application of sewage sludge increases the abundance of antibiotic resistance genes in soil. Environ. Int. 92–93, 1–10.
Cycoń, M.; Mrozik, A.; Piotrowska-Seget, Z. (2019) Antibiotics in the Soil Environment—Degradation and Their Impact on Microbial Activity and Diversity. Front. Microbiol. 10, 338.
Du, L.; Liu, W. (2011) Occurrence, fate, and ecotoxicity of antibiotics in agro-ecosystems. A review. Agron. Sustain. Dev. 32, 309–327.
Economou, V.; Gousia, P. (2015) Agriculture and food animals as a source of antimicrobial-resistant bacteria. Infect. Drug Resist. 8, 49–61.
Gorovtsov, A.V.; Sazykin, I.S.; Sazykina, M.A. (2018) The influence of heavy metals, polyaromatic hydrocarbons, and polychlorinated biphenyls pollution on the development of antibiotic resistance in soils. Environ. Sci. Pollut. Res. 25, 9283–9292.
Gudda, F.O.; Waigi, M.G.; Odinga, E.S.; Yang, B.; Carter, L.; Gao, Y. (2020) Antibiotic-contaminated wastewater irrigated vegetables pose resistance selection risks to the gut microbiome. Environ. Pollut. 264, 114752.
He, J.; Yan, Z.; Chen, Q. (2020) Transmission of antibiotic resistance genes in agroecosystems: An overview. Front. Agric. Sci. Eng. 7, 329–332.
Iwu, C.D.; Korsten, L.; Okoh, A.I. (2020) The incidence of antibiotic resistance within and beyond the agricultural ecosystem: A concern for public health. MicrobiologyOpen 9, e1035.
Jechalke, S.; Heuer, H.; Siemens, J.; Amelung, W.; Smalla, K. (2014) Fate and effects of veterinary antibiotics in soil. Trends Microbiol. 22, 536–545.
Jin, L.; Xie, J.; He, T.; Wu, D.; Li, X. (2021) Airborne transmission as an integral environmental dimension of antimicrobial resistance through the “One Health” lens. Crit. Rev. Environ. Sci. Technol. 52, 4172–4193.
Kabelitz, T.; Ammon, C.; Funk, R.; Münch, S.; Biniasch, O.; Nübel, U.; Thiel, N.; Rösler, U.; Siller, P.; Amon, B.; et al. (2020) Functional relationship of particulate matter (PM) emissions, animal species, and moisture content during manure application. Environ. Int. 143, 105577.
Marshall, B.M.; Levy, S.B. (2011) Food Animals and Antimicrobials: Impacts on Human Health. Clin. Microbiol. Rev. 24, 718–733.
Mathews, S.; Reinhold, D. (2013) Biosolid-borne tetracyclines and sulfonamides in plants. Environ. Sci. Pollut. Res. 20, 4327–4338.
Münch, S.; Papke, N.; Thiel, N.; Nübel, U.; Siller, P.; Roesler, U.; Biniasch, O.; Funk, R.; Amon, T. (2020) Effects of farmyard manure application on dust emissions from arable soils. Atmospheric Pollut. Res. 11, 1610–1624.
Pan, M.; Chu, L. (2017) Fate of antibiotics in soil and their uptake by edible crops. Sci. Total. Environ. 599, 500–512.
Tadić, D.; Hernandez, M.J.B.; Cerqueira, F.; Matamoros, V.; Piña, B.; Bayona, J.M. (2021) Occurrence and human health risk assessment of antibiotics and their metabolites in vegetables grown in field-scale agricultural systems. J. Hazard. Mater. 401, 123424.
Wang, F.; Fu, Y.-H.; Sheng, H.-J.; Topp, E.; Jiang, X.; Zhu, Y.-G.; Tiedje, J.M. (2021) Antibiotic resistance in the soil ecosystem: A One Health perspective. Curr. Opin. Environ. Sci. Health 20, 100230.
Wang, J.; Wang, L.; Zhu, L.; Wang, J.; Xing, B. (2020) Antibiotic resistance in agricultural soils: Source, fate, mechanism and attenuation strategy. Crit. Rev. Environ. Sci. Technol. 52, 847–889.
Wang, F.-H.; Qiao, M.; Chen, Z.; Su, J.-Q.; Zhu, Y.-G. (2015) Antibiotic resistance genes in manure-amended soil and vegetables at harvest. J. Hazard. Mater. 299, 215–221.
Zalewska, M.; Błażejewska, A.; Czapko, A.; Popowska, M. (2021) Antibiotics and Antibiotic Resistance Genes in Animal Manure–Consequences of Its Application in Agriculture. Front. Microbiol. 12, 610656.
Zhao, F.; Yang, L.; Chen, L.; Li, S.; Sun, L. (2018) Bioaccumulation of antibiotics in crops under long-term manure application: Occurrence, biomass response and human exposure. Chemosphere 219, 882–895.
Zhang, T.; Li, X.; Wang, M.; Chen, H.; Yang, Y.; Chen, Q.-L.; Yao, M. (2019) Time-resolved spread of antibiotic resistance genes in highly polluted air. Environ. Int. 127, 333–339.