Background Knowledge
Soil functions
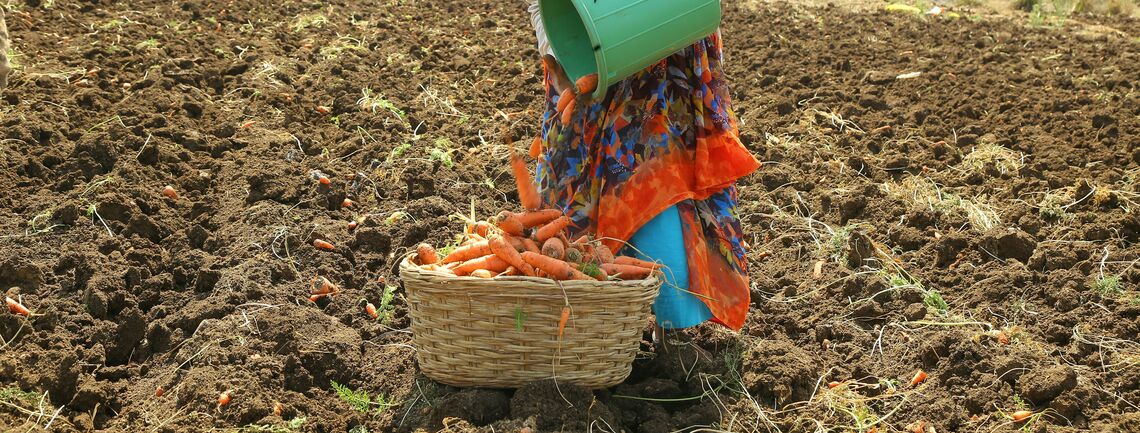
Soil functions are dynamic soil properties that emerge from complex interacting biogeophysical processes within the soil and that are relevant for human well-being. They are the basis for soil related ecosystem services and resource use efficiency. The concept of soil functions highlights the relevance of soils for human societies and facilitates their structured scientific assessment.
Soil functions first gained prominence through the proposed European Soil Thematic Strategy (EU 2006). For agricultural soils, five functions are considered particularly relevant (Schulte et al., 2014; Helming et al., 2018; Vogel et al., 2018):
Production of plant biomass
Storing and filtering of water
Storing and recycling of nutrients
Habitat provision
Carbon storage
According to the cascade model of ecosystem services (Haines-Young & Potschin, 2010), soil functions are bundles of soil-based, biophysical-chemical structures and processes with the capacity to provide services to human societies (de Groot et al., 2010). They can be seen as the basis for soil related ecosystem services, but also for soil related resource-use efficiency. Soil functions first gained prominence through the proposed European Soil Thematic Strategy (EU, 2006) where they represented soils’ contribution to: food, fibre and biomass provision, filtering, storing and accumulating of water nutrients and carbon, providing habitats and preserving gene pools, providing a platform for human activities, and acting as a historical archive. In an agricultural context, the functions as a platform for activities or as a historical archive are usually not considered relevant, whereas the functions pertaining to water, nutrients and carbon are of the highest importance.
Consequently, Vogel et al. (2018) define the five (agricultural) soil functions listed below. The BonaRes project uses their definition and describes the functions in the following way:
Biomass production: “Soils are indispensable for plant growth and herewith for the production of biomass, which can be used as food supply for humans and animals but also as raw material or energy source. The optimization of crop yields under strongly varying external conditions (temperature, precipitation frequency and intensity, etc.) is one of the biggest challenges with respect to biomass production. Soil fertility is linked to its non-rigid porous structure allowing for roots to penetrate, to store water while air and oxygen is also available and to retain nutrients to be used by plants and recycled by an enormous diversity of soil biota.
Deficiencies in one or more of these conditions might be compensated by agricultural measures – e.g. compaction by tillage, missing nutrients by fertilization – on the long run, however, sustainable biomass production needs to rely on the natural capacity of soil to provide these essential features.”
Storage and recycling of nutrients: “The cycling of macro- and micronutrients is an essential function of soils. Mineral weathering generates a slow but constant source of elements made available for biochemical cycles. Soils are biochemical reactors, where numerous organic and inorganic compounds are decomposed and transformed so that the nutrients become again available for soil biota and biomass production. The engine driving this reactor is the myriad of organisms living in soil and taking profit from the energy stored in plant residues. Some part of it, however, is protected from decomposition for quite a long time so that soils act as a substantial carbon pool. An important feature is the ability of soil to retain nutrient within the root zone and to avoid leaching towards groundwater or emission to the atmosphere where the same nutrients might be harmful (e.g. NO3 in groundwater or N2O in the atmosphere).”
Carbon sequestration: “Globally, there is more carbon in soils than in the atmosphere and the aboveground biomass together. Plants extract carbon dioxide from the atmosphere by photosynthesis and incorporate it in their cell structures. This carbon then enters the soil as root exudates from living plants, which are utilized by soil organisms, or by decomposition of plant litter. These processes release carbon dioxide back to the atmosphere. They are more or less rapid depending on climate, soil type, land use, and management practices. Since carbon dioxide is a greenhouse gas, increasing soil organic matter stocks in soil is considered as a possible solution to mitigate climate change. Soil organic matter has also beneficial effects on soil fertility, water retention, susceptibility to erosion, and diversity and activity of soil organisms.”
Habitat for biological activity: “There are more species living below ground than above. Soils provide the living space for an enormous diversity of organisms, but in turn, are also formed by soil biota. The activity and diversity of soil organisms are largely affected by biotic and abiotic factors (e.g., climate, soil properties), but also by land use and management practices. Soil organisms play important roles in a number of soil processes such as nutrient cycling, mixing of soil material and development of soil structure. Due to the high diversity of soil organisms, many species are thought to be redundant, which means that they can be replaced by other species fulfilling the same function. However, certain species may play an irreplaceable role in soil functions. Soil structure provides a multitude of niches where different species can coexist with reduced competition for food and reduced stress of being caught by predators. This is a fundamental difference to the living space above ground.”
Filtering and storage of water: “Depending on soil properties, precipitation water is partitioned into storage, groundwater recharge, evapotranspiration, and surface runoff. Due to its porous structure, water can infiltrate into soil and is retained there via capillary forces just like in a sponge, so that it is available for plant growth and the development of other organisms. This reduces droughts and the generation of floods. Solutes and particles moving through soil have to pass the extensive inner surfaces of soil constituents where they might be sorbed or transformed. Hence, soils are also important to filter harmful substances, originating from industrial and agricultural production, municipal activities, or atmospheric deposits.”
Please note: Each of these functions is a collective term with numerous sub-functions. For example, the function “storing and recycling of nutrients” comprises individual functions for the storage of all macro- and micro-nutrients, as well as functions relevant for the transformation of the different molecules containing these nutrients.
The importance of managing soil functions to support ecosystem services is widely acknowledged (Glaesner et al., 2014; Schulte et al., 2014; Breure et al., 2012). They represent the biogeophysical reality that is affected by agricultural management. Sustainable intensification, beyond a simple increase or reduction of management intensity, can only be achieved if management can be adjusted to soil internal processes in order to optimize the provision of soil functions. Higher provision of soil functions would then in turn lead to a higher provision of ecosystem services and a higher resource use efficiency.
Soil functions are part of the overall category of ecosystem functions and as such, closely related to ecosystem services. Ecosystem services, the “direct and indirect contributions of ecosystems to human well-being” (Kumar, 2010), are based on the use or appropriation of ecosystem functions (Boyd & Banzhaf, 2007). The underlying terminology builds on the cascade model by Potschin & Haines-Young (2011), where biophysical function or structure, ecosystem function, ecosystem service, benefit and (economic) value are connected elements along a cascade-chain (TEEB, 2010; Braat & de Groot, 2012; Haines-Young & Potschin, 2010; Potschin-Young et al., 2017). By definition, impacts analysed in an impact assessment occur at intersection between the biophysical and the human sphere. Because soil functions fully belong to the biophysical sphere, they are themselves not considered to be impacts. Rather, they are the biophysical basis for impacts such as ecosystem services and resource use efficiency.
However, the operationalisation of linkages between soil management, soil functions, and ecosystem services (Helming et al., 2018; Stavi et al., 2016; Schwilch et al., 2016) and resource use efficiency (Helming et al., 2018) remains a challenge. To address these linkages, a clearly defined terminology is imperative. Unfortunately, the hierarchical relationship between soil functions and ecosystem services is not as straight-forward as it should be, and there is an overlap in definitions as discussed by Baveye et al. (2016). Consequently soil function and soil related ecosystem service are often not clearly distinguished in current literature (an exception is e.g., Pulleman et al., 2012, investigating soil biodiversity). The overlap in definitions is particularly evident for the production of biomass (a soil function) and the provision of plant-based food, fibres and energy (an ecosystem services).
With the BonaRes Assessment Platform we will focus on soil-based ecosystem services and resource use in order to make the benefits of soils for human well-being visible and to inform sustainable soil management and policy. This also facilitates the integration of soil related impact assessments into a wider societal context. For the assessments we draw on biogeophysical information provided by the soil functions and their respective sub-functions. The individual linkages are highlighted in the sections on Ecosystem Services and Resource Use Efficiency.
Baveye P C, Baveye J, Gowdy J. 2016. Soil “ecosystem” services and natural capital: Critical appraisal of research on uncertain ground. Frontiers in Environmental Science 4: 41. DOI:10.3389/fenvs.2016.00041
Boyd J, Banzhaf S. 2007. What are ecosystem services? The need for standardized environmental accounting units. Ecological Economics 63: 616–626. DOI:10.1016/j.ecolecon.2007.01.002
Braat L C, de Groot R. 2012. The ecosystem services agenda: bridging the worlds of natural science and economics, conservation and development, and public and private policy. Ecosystem Services 1: 4-15. DOI:10.1016/j.ecoser.2012.07.011
Breure A M, de Deyn G B, Dominati E, Eglin T, Hedlund K, van Orshoven J, Posthuma L. 2012. Ecosystem services: a useful concept for soil policy making! Current Opinion in Environmental Sustainability 4: 578–585. DOI:10.1016/j.cosust.2012.10.010
de Groot R S, Alkemade R, Braat L, Hein L, Willemen L. 2010. Challenges in integrating the concept of ecosystem services and values in landscape planning, management and decision making. Ecological Complexity 7: 260–272. DOI:10.1016/j.ecocom.2009.10.006
EU – European Union. 2006. EU Soil Thematic Strategy. COM(2006)231 final.
Glaesner N, Helming K, de Vries W. 2014. Do current European policies prevent soil threats and support soil functions? Sustainability 6(12): 9538-9563. DOI:10.3390/su6129538
Haines-Young R, Potschin M. 2010. The links between biodiversity, ecosystem services and human well-being. In: Raffaelli DG, Frid C (Eds.): Ecosystem Ecology: A New Synthesis. BES Ecological Reviews Series, Cambridge University Press, Cambridge, New York, pp. 110–139.
Helming K, Daedlow K, Paul C, Techen AK, Bartke S, Bartkowski B, Kaiser D, Wollschläger U, Vogel HJ. 2018. Managing soil functions for a sustainable bioeconomy—Assessment framework and state of the art. Land Degradation & Development 29: 3112–3126. DOI:10.1002/ldr.3066
Kumar P (Ed.). 2010. The Economics of Ecosystems and Biodiversity: Ecological and Economic Foundations. Routledge, London; New York.
Potschin M, Haines-Young R. 2011. Ecosystem Services: Exploring a geographical perspective. Progress in Physical Geography 35(5): 575-594. DOI:10.1177/0309133311423172
Potschin-Young M, Haines-Young R, Görg C, Heink U, Jax K, Schleyer C. 2017. Understanding the role of conceptual frameworks: Reading the ecosystem service cascade. Ecosystem Services 29(C): 428-440. DOI:10.1016/j.ecoser.2017.05.015
Pulleman M, Creamer R, Hamer U, Helder J, Pelosi C, Pérès G, Rutgers M. 2012. Soil biodiversity, biological indicators and soil ecosystem services—an overview of European approaches. Current Opinion in Environmental Sustainability 4: 529–538. DOI:10.1016/j.cosust.2012.10.009
Schulte R P O, Creamer R E, Donnellan T, Farrelly N, Fealy R, O’Donoghue C, O’Huallachain D. 2014. Functional land management: A framework for managing soil-based ecosystem services for the sustainable intensification of agriculture. Environmental Science & Policy 38: 45–58. DOI:10.1016/j.envsci.2013.10.002
Schwilch G, Bernet L, Fleskens L, Giannakis E, Leventon J, Marañón T, Mills J, Short C, Stolte J, van Delden H, Verzandvoort S. 2016. Operationalizing ecosystem services for the mitigation of soil threats: A proposed framework. Ecological Indicators 67: 586–597. DOI:10.1016/j.ecolind.2016.03.016
Stavi I, Bel G, Zaady E. 2016. Soil functions and ecosystem services in conventional, conservation, and integrated agricultural systems. A review. Agronomy for Sustainable Development 36: 32. DOI:10.1007/s13593-016-0368-8
TEEB (The Economics of Ecosystems and Biodiversity). 2010. Mainstreaming the Economics of Nature: A Synthesis of the Approach, Conclusions and Recommendations of TEEB.
Vogel HJ, Bartke S, Daedlow K, Helming K, Kögel-Knabner I, Lang B, Rabot E, Russell D, Stößel B, Weller U, Wiesmeier M, Wollschläger U. 2018. A systemic approach for modeling soil functions. SOIL 4: 83–92. DOI:10.5194/soil-4-83-2018